How to observe a quantum wave packet in situ?
Researchers have successfully observed the free expansion of a quantum wave packet of a single atom in continuous space. Using a novel imaging technique that combines optical trapping and quantum gas microscopy, they tracked with unprecedented precision the spreading of the probability density associated with an atom released from its trap.
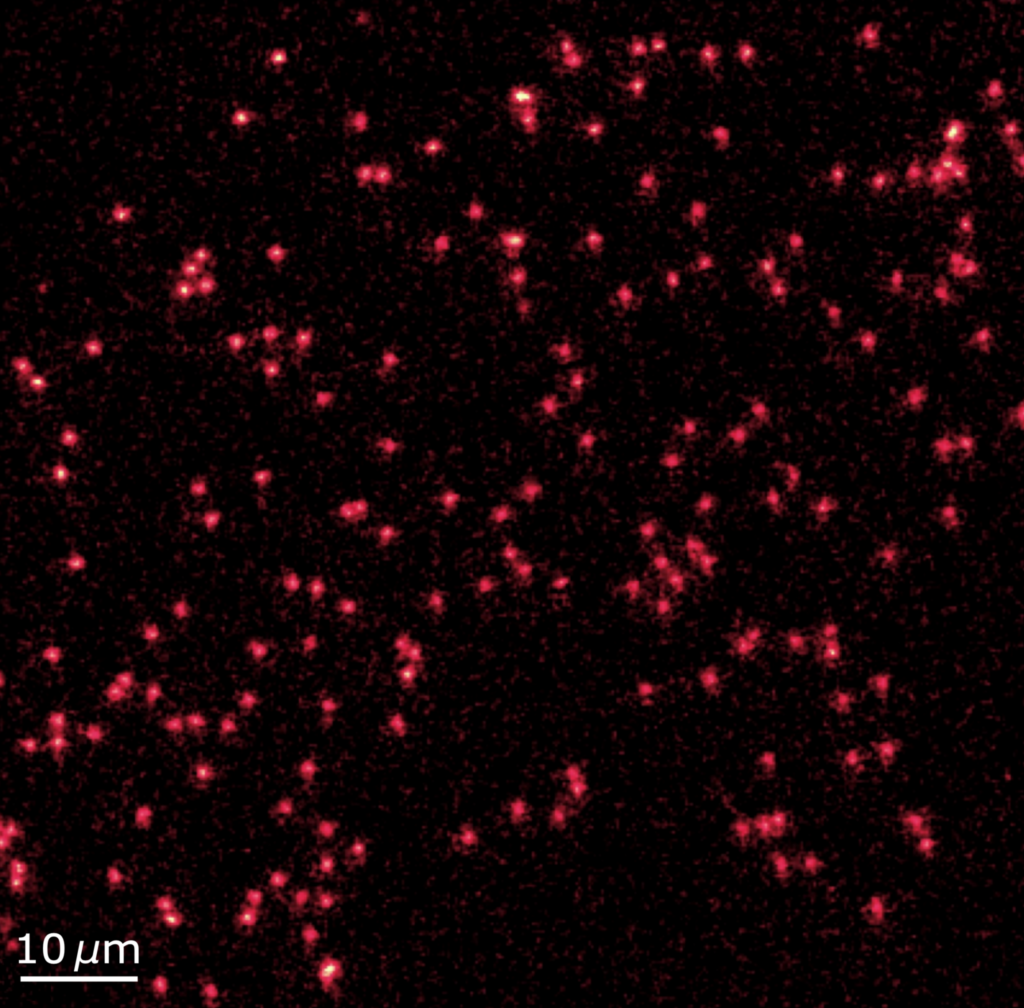
Each red glowing dot corresponds to the fluorescence signal of a single lithium atom trapped in an optical lattice. © Joris Verstraten et al., 2024.
Since Louis de Broglie’s work in 1924, the concept of wave-particle duality has been central to quantum mechanics. When an atom is confined in a trap, its wavefunction is localized much like a particle. But once released (by turning off the trap), the wavefunction progressively spreads out in space, following an evolution predicted by the famous Schrödinger equation. Directly observing the dynamics of such an object in free space represents a major experimental challenge, one that could reveal fundamental properties of many quantum systems.
Until now, quantum gas experiments with single-atom resolution — a technique known as quantum gas microscopy — were limited to ensembles of atoms evolving within a discrete structure made of spatially periodic traps, preventing the study of continuous quantum systems.
This research was carried out in the following laboratories:
- Laboratoire Kastler Brossel (LKB, CNRS/Collège de France/ENS-PSL/Sorbonne Université)
- Laboratoire Collisions Agrégats Réactivité (LCAR, CNRS/Université de Toulouse)
A recent study paves the way for quantum gas microscopy in continuous space. In their experiment at the Laboratoire Kastler Brossel, researchers first prepared wave packets of individual lithium atoms confined in the potential wells of an optical lattice. Once released into a plane where they could evolve freely, these atoms were recaptured using a projection method into a deep optical lattice, allowing for precise reconstruction of their motion. By repeating this experiment across many atoms, the researchers were able to reconstruct the spatio-temporal evolution of the wavefunction with unprecedented fidelity.
This study establishes a protocol to project an atom from continuous space onto the nearest lattice site in a controlled way, with fidelity exceeding 99%, laying the foundation for continuous-space quantum gas microscopy. The imaging technique developed here can be likened to a “CCD sensor” for atomic wavefunctions.
Beyond this experimental breakthrough, the new method for imaging individual atoms developed by the research team opens the door to exploring more complex quantum systems in continuous space. The technique is already being used by the team to investigate strongly correlated fermionic systems at the microscopic scale — systems that are notoriously difficult to tackle theoretically.
These results are published in Physical Review Letters.
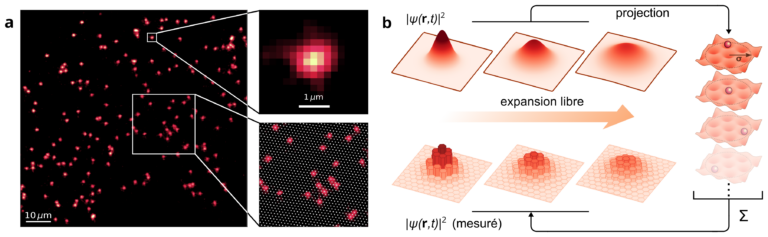
(b). Experimental protocol used to measure the free expansion of the wave packet of individual atoms. After expansion, the optical lattice is suddenly switched on, projecting the atoms from continuous space onto the lattice sites. In each experimental realization, the atom is localized on a different site, but the probability density |ψ(r,t)|² can still be reconstructed by averaging the positions over many repetitions. The imaging method developed here — quantum gas microscopy in continuous space — can be likened to a “CCD sensor” for atomic wavefunctions.
© Joris Verstraten et al., 2024.