Research topics
Testing Quantum ElectroDynamics with atomic hydrogen/deuterium
Charge Radius Experiment with Muonic Atoms
Probing short range forces with gravitationnal quantum states of light particles
Testing Quantum ElectroDynamics with atomic hydrogen/deuterium
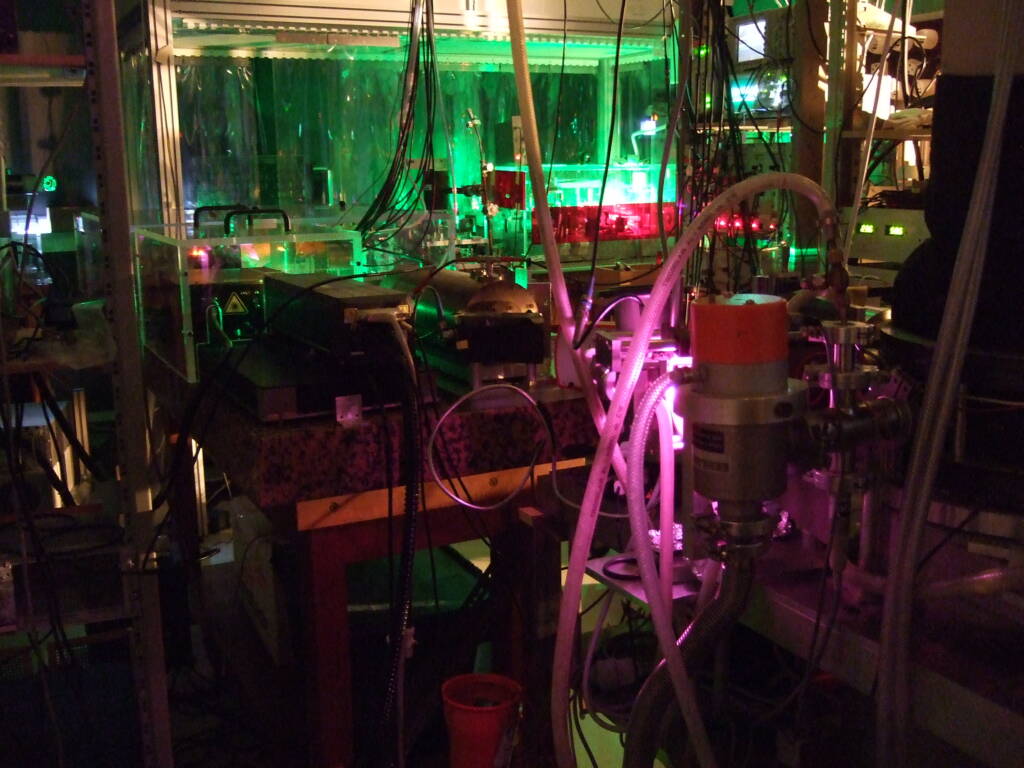
Atomic hydrogen is the simplest atom. Theoretical and experimental studies of its energy levels have led and still lead to numerous advances in quantum mechanics.
In our group, we carry out high-resolution spectroscopy of hydrogen (deuterium) with continuous lasers. Their frequencies are measured with an optical frequency comb referenced to an ultra-stable laser signal generated at the SYRTE laboratory and delivered to our laboratory by the REFIMEVE fiber-optic network. Today, the theory-experiment comparison allows us to test theory at the level better than the ppt (10-12).
Charge Radius Experiment with Muonic Atoms
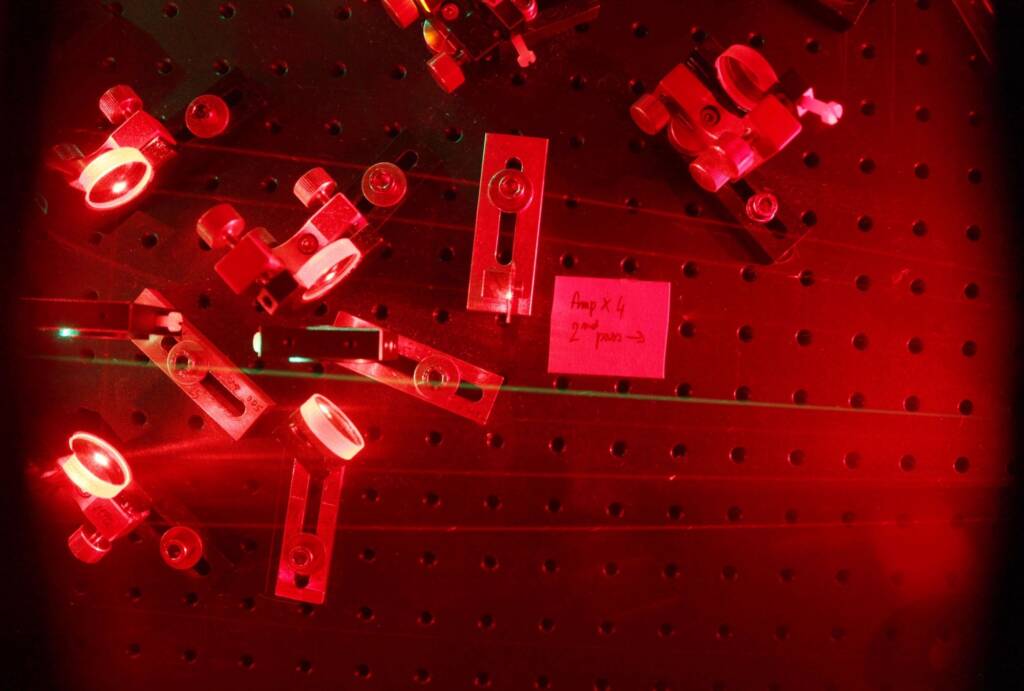
Atomic nuclei are composed of protons and neutrons. In atoms, electrons orbit around these nuclei. These particles (protons, neutons, electrons) are the main components of matter on earth. Muonic atom is an atom in which an electron has been replaced by a muon. With the same electrical charge, the muon is 207 times heavier than an electron. Therefore, muonic atom has a correspondingly smaller size than its electronic counterpart, making it more sensitive to the electrical and magnetic properties of the nucleus. These properties are determined by laser spectroscopy of the muonic atom. The experiments carried out by an international collaboration CREMA, takes place at the Paul Scherrer Institute (Switzerland), where the muon source is located. Having determined the electrical charge distribution of the proton (deuteron, helion and alpha particles) with the greatest precision ever achieved, the current experiment aims to probe the proton’s magnetic properties.
Probing short range forces with gravitationnal quantum states of light particles
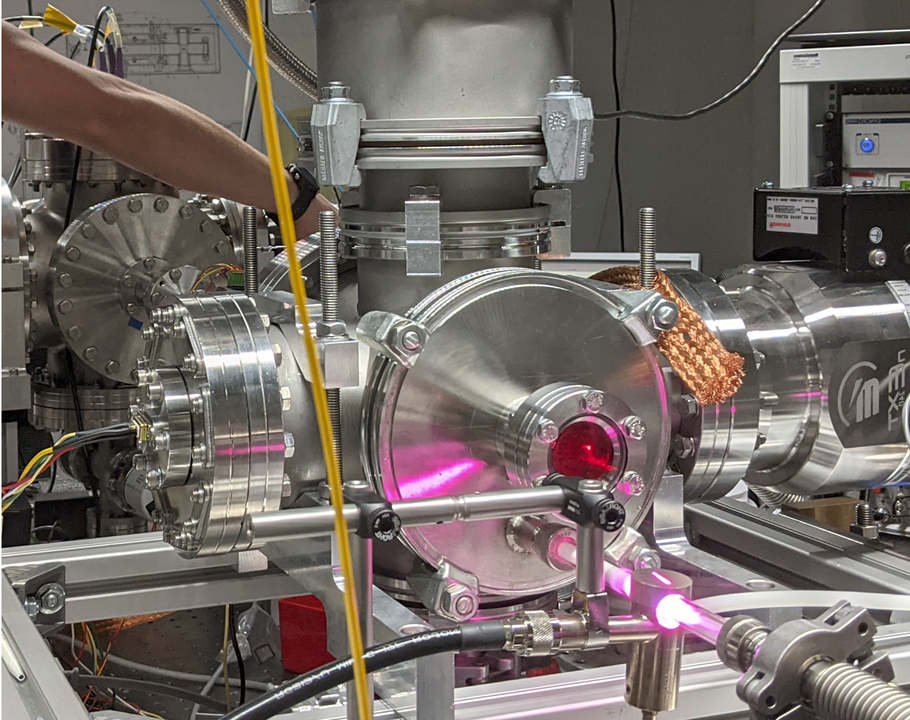
In this topic, we aim to explore the boundaries of fundamental law of physics in an unexplored parameter range by studying quantum properties of light neutral particles (hydrogen atoms, neutrons) in the field of gravity, Casimir-Polder and Van-der-Waals potentials.
The necessary long interaction time between particles, gravity and potentials is achieved when particles are in gravitational quantum states. These states are realized with light particles trapped in the gravitational field (top confinement), bouncing onto a surface thanks to quantum reflections (bottom confinement). Quantum reflections are driven by the surface’s potential and the vertical particle’s velocity.
Currently, sources of hydrogen atoms with low velocities, as well as novel techniques for their trapping and detection are developed in Turku (Finland) and Vienna (Austria). Then we aim to measure for the first time quantum effects of gravity in a Bose Einstein condensate, gravitational quantum states of atoms and discrete shifts of optical and hyperfine transitions in hydrogen bound in gravitational quantum states. This work is done within the international collaboration GRASIAN (https://grasian.eu) created in 2019.